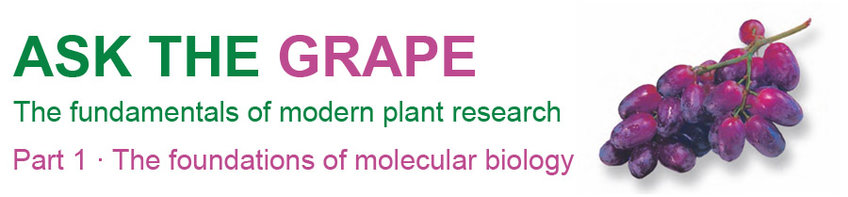
Why are researchers interested in the genetic material of plants?
Nothing functions without genes
All of the traits of an organism, regardless of whether it is a plant, animal, or human, are stored in the genetic material in the form of genes and are expressed in accordance with the environmental conditions. They work alone or in groups. The complete set of genes of an organism (genome) thus constitutes the “operating manual” for the composition of characteristics of a living being. It is responsible for the formation of substances at the right time and for the correct execution of all processes in an organism. Modern techniques now allow scientists to carry out their research on the level of the genetic material. Scientists no longer just observe and study individual processes and reactions, but now examine and analyze larger interdependent processes as well as their interactions with the environment. Their goal is to trace and understand the growth, development, and metabolism of plants. If these basics can be understood, in a next step they can be used to increase the effectiveness of breeding, to practice sustainable agriculture, and to make predictions about the impact of environmental factors on plants.
What are traits?
Color, taste, toxicity – all a question of genes
A trait describes a particular property of an organism, like, e.g., flower color. Many traits are externally visible. Others, like for example, taste or toxicity cannot be recognized from the outside. These can only be determined through a compositional analysis. The entire set of traits, whether they are externally visible or not, is termed the phenotype.
Genotype denotes the genetic make-up of an organism, which is the basis for all traits. The development of traits requires that a gene be switched on and read (see the “How do genes determine traits?” chapter).
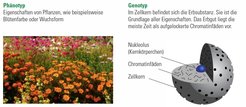
What are qualitative or quantitative traits?
Not all traits are the same
The expression of some organismal traits is clearly defined and apparent, like for example, leaf shape or flower color. Such properties, that are controlled by only one or from very few genes and are hardly influenced by the environment, are known as qualitative traits. These were the traits observed by Mendel in his experiments. This allowed him to take note of rules and to formulate Mendel’s laws (Ask the Plum).
Other properties, in contrast, are not easily defined, such as plant size or biomass. Such properties are known as measurable properties. These are generally determined by several genes and can be further influenced by the environment to greater or lesser extents. In plant breeding, it is thus difficult to select the right parents for these traits and to make clear predictions regarding the composition of traits of the children or to choose, at an early stage, the most promising progeny. New insights and methods can help in selecting “the best” and in shortening the time required for breeding processes (Ask the Grape, Part 2 - coming soon).
What is the hereditary material made of?
The structure behind the trait
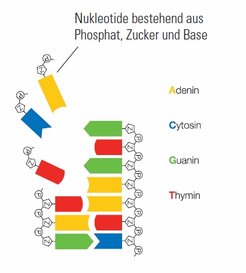
The genetic material is also known as deoxyribonucleic acid (abbreviated as DNA) and carries the information for all the traits of an organism. The traits of all living beings are based on only four bases: adenine (A), cytosine (C), guanine (G), and thymine (T). Base, phosphate, and sugar components together form a nucleotide. The bases, or rather the nucleotides, are ordered one after another in a long chain in molecules of DNA.
DNA does not exist as a single band of nucleotides but rather as a double strand. The so-called backbone is formed by sugar and phosphate on the outside of the strand. The bases of the two strands pair with each other in the middle. Because of their different structures not all bases can face each other. Only adenine and thymine as well as cytosine and guanine fit together. If the base sequence of one strand is known, this automatically yields the sequence in which the bases on the partner strand are ordered. The strands are complementary to each other.
Specific segments form an information unit. A new unit of information – gene – begins and also ends with a particular sequence of bases. In this way, it is clear during the reading process from where to where a gene stretches. A large number of bases lie between the start and end points; the sequence of these bases determines the trait. In front of, as well as partly downstream of this sequence, are found segments that are important for regulating the reading process of a gene. In addition, the information-carrying areas (exons) are often interrupted by interposing segments (introns). Previously, it was assumed that these had no function (“junk DNA”). Today it is known that they can exert an influence on the reading process.
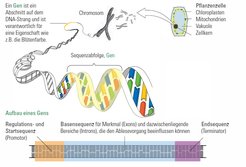
How are chromosomes, DNA, and genes linked together?
The who’s who of heredity
Chromosomes, found in the nucleus of every single cell of every living being, consist of DNA and proteins. Genes are located on the DNA in individual segments. Depending on the phase, a chromosome can possess one or two “arms” called chromatids. Every chromatid possesses a double strand of DNA, although most of the time nuclei contain individual chromatids. Only before mitosis – cell division – are chromatids duplicated so that chromosomes are formed, which possess two identical chromatids. The same process also occurs shortly before reduction division, the first cell division in meiosis.

Most of the time, chromosomes in the nucleus are filamentous 1-chromatids. The typical x-shaped chromosomes are just visible shortly before or rather during cell division (mitosis) or rather before the reduction division (meiosis). For the rest of the time, chromosomes exist as diffuse relaxed 1-chromatid chromosomes.
Why do children differ from their parents?
Variability through recombination and chance
Children often look like their parents. Sometimes a little more like the father, sometimes a little more like the mother, and sometimes it is hard to determine any similarities at all. Why is this the case and how can it be explained?
The reason for this is that children, whether human, animal, or plant, inherit properties from their fathers as well as from their mothers. In organisms with a double set of chromosomes, the children receive one set of chromosomes from the father and one from the mother. To avoid the addition of chromosome sets at every fertilization and to keep the number always the same, during gamete formation chromosome number is first halved in a process called meiosis. Chromosomes previously originating from the father and mother are divided in such a way that each gamete only receives one chromosome from each chromosome pair.
During this process, chromosome arms (chromatids) from the maternal and paternal chromosomes may overlap. At these points of overlap, breaks can occur and the chromatids may then be put back together in a cross-wise manner, so that the maternal chromosome receives a part of the paternal chromosome and vice versa. This process is called crossing over. At the end of this phase, two cells have been formed that both harbor a single chromosome set and recombined chromosomes. In the next step, the two arms of the chromosome are separated from each other, so that the genetic material is eventually randomly divided among four daughter cells.

Seperation of the homologous chromosomes
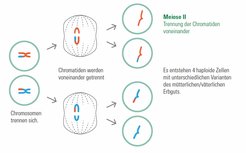
Seperation of the Chromatids
Egg and sperm cells – that is the gametes from the father and mother – meet during fertilization and pair with each other. This means that the fertilized egg contains one chromatid from the mother and one from the father. As a result of the processes occurring during meiosis, the sperm cells formed by the father are not identical, and their genetic composition varies. The same is true for the egg cells produced by the mother. Depending on which sperm cell encounters which egg, the offspring may differ greatly from each other. The variations only occur in the genetic material available to both the mother and father, however. This is why, on the one hand, children differ from their parents, but, on the other hand, share similarities with both.
What happens during growth?
Every cell needs the same information
After a successful fertilization, an embryo grows, meaning that the cells reproduce themselves. In this process, the nucleus of a cell divides and two identical daughter cells are formed with exactly the same genetic information. How does this process, called mitosis, proceed?
To begin, duplication of the maternal and paternal chromatids again leads to the formation of a chromosome with two arms or, in scientific terms a duplicated or replicated chromosome. Then, in a process called mitosis, the chromatids of the chromosomes are divided, so that in a newly formed cell in organisms with a so-called diploid chromosome set there is again a double set of chromosomes: one from the father and one from the mother (initially again as non-replicated chromosomes possessing only one chromatid).
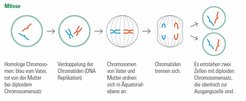
One cell results in two identical daughter cells.
What are mutations and how do they occur?
Variability through mistakes
Sexual reproduction and cross-pollination (Ask the Barley) result in progeny that exhibit a mixture of the parental characteristics. Unscheduled, and partly far-reaching changes to DNA are known as mutations. They lead to children with properties that are different to those that were possessed by their parents. Causes of mutation include mistakes during the duplication of chromatids or the halving of the chromosome set. An incorrect base may be incorporated into DNA, a base may even be forgotten, or a chromosome may be present in three copies instead of two.
Mutations can also be triggered by physical factors such as UV radiation, radioactivity, or chemicals. This has been taken advantage of in plant breeding in the past to furnish plants with new properties.
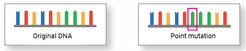
The example of point mutations: One base is replaced by another. This can potentially lead to a change in the sequence of amino acids in the protein (see the “How are proteins formed” chapter), which can result in a change or loss of function of the protein. However, as not all DNA codes for proteins, changes may also occur that do not show any effects.
Why are mutations important?
Adaptation through change

Mutations are one of the most important factors in evolution. They can result in an advantage for the respective organism, in which case they will as a rule prevail, or they can result in a disadvantage and can lead to death in the worst-case scenario, if, for example, vital metabolic pathways are affected. Likewise, there are so-called silent mutations that have neither positive nor negative consequences, as well as neutral mutations that produce changes but have no significance for the survival of the organism. Nowadays, methods of genetic engineering offer, in contrast to mutations, the possibility of introducing new properties to plants in a targeted manner.
Plants that do not engage in sexual reproduction and thus give rise to progeny with identical compositions of traits, can also be changed by mutations, so that also here different variants and adaptations can emerge.
How do genes determine traits?
Proteins are not only there to be eaten
While the DNA-localized genes are the carriers of the genetic information, proteins are the important executors of function within an organism. The type, composition, and structure of proteins largely determine the properties of an organism and ensure that plants grow and develop. There is a whole range of proteins with different functions. Certain proteins form structural polymers for tissues and cells, others are responsible for the regulation of metabolic processes, others control growth and maturation, while still others function to transport substances or to protect against diseases and pests. Proteins are assembled from chains of amino acids that are folded into specific spatial configurations.
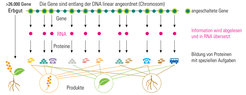
How are proteins formed?
Copying, transport, and translation

As the genetic information is very valuable, it cannot be damaged under any circumstances. For this reason, DNA also remains in the nucleus. However, as protein synthesis takes place in the cellular cytoplasm, a form of transport known as messenger RNA or mRNA is used. Depending on what proteins the cell needs at a given time, particular regions of the DNA (switched-on genes) are read on the non-replicated chromosome and converted into mRNA. This process is called transcription. RNA is also comprised of a sequence of nucleotides that consist of a phosphate, a sugar (ribose), and a base. As in DNA, four different bases are used; three of these are identical to those of DNA (adenine, guanine, and cytosine), while the fourth RNA base is called uracil. A unit (triplet) is always composed of three bases which together code for one amino acid.
According to requirements, mRNA can be produced and degraded and is transported from the nucleus into the cytoplasm. This is where large enzyme complexes, called ribosomes, bind to mRNA. The amino acids are attached to transfer RNA or tRNA when they enter the ribosome; complementary bases on tRNA bind to bases on mRNA. In the ribosomes, the individual amino acids are linked together to form proteins. This conversion from the language of nucleotides (mRNA) into the language of the amino acids (proteins) is called translation.
Not all of the genome (the entire DNA of an organism) is translated into proteins and large areas do not code for proteins at all. These sections of DNA were previously known as “junk DNA” asno function could be ascribed to them. Today we know that these non-coding regions also perform tasks. For example, they control when a gene is read or which parts of the gene are translated into proteins.
What do proteins look like?
Structure determines function
Proteins are macromolecules that are assembled from amino acids. In protein biosynthesis, the amino acids are linked together with peptide bonds to form chains.
Proteins are found in all cells and carry out important functions. Their spatial configuration determines their mode of action. A distinction is made between the primary structure, which describes the sequence of amino acids in a polypeptide chain, and the secondary structure, that is formed by interactions between the side chains of the participating amino acids. These helical or sheet-like structures can be folded into a higher-level spatial structure called the tertiary structure. Last but not least, several proteins can join together in protein complexes, which is then termed the quaternary structure. The spatial configuration of proteins is based on the identity of the amino acids involved, which is in turn determined by the genetic code. Gene mutations, mistakes during transcription or translation, as well as environmental factors can lead to changes in the primary structure and thereby to mistakes in folding. This can result in the loss of the protein’s function.