Systems and Synthetic Metabolism
The Group “Systems and Synthetic Metabolism” studies the biochemical logic and design principles of metabolic pathways and their applications in metabolic engineering of microbes. The group focuses on engineering synthetic alternatives to central metabolic pathways, aiming to uncover optimality in metabolic designs and to offer novel solutions for humanity’s needs in chemical and energy production.
In recent years we have witnessed a renaissance in metabolic research. Many current research efforts attempt to address emerging grand challenges in sustainable energy, green chemistry and pharmaceuticals by tinkering with central and secondary metabolism. Deep understanding is essential for all these efforts: in order to study, manipulate or redesign metabolism one must gain a solid grasp of the biochemical principles governing it.
Why metabolic pathways are structured the way that they are? Which biochemical constraints are responsible for the fact that different organisms use different pathways? Is the conservation of metabolic pathways throughout the tree of life a sign of their optimality, or rather an indication of a frozen accident taking place near the origin of life? We try to tackle these questions by studying, computationally and experimentally, the different components of metabolic pathways (enzymes, metabolites, etc.) and the different elements that affect their function (thermodynamics, kinetics, stability, reactivity, etc.)
Engineering novel routes for one-carbon metabolism
Modern society is a ravenous consumer of commodities and energy as well as perpetual source of superfluous byproducts release to the environment. As such, three of the main global challenges our society faces are the production and storage of renewable energy, identification of new highly available feedstocks for the production of everyday commodities, and development of effective methods to reduce the concentration of byproducts in the environment, especially atmospheric CO2. Addressing these challenges requires unconventional approaches that integrate physics, chemistry and biology.
One carbon compounds offer a unique opportunity to tackle these global challenges. Formic acid is especially interesting, as it can be efficiently produced from various available resources including electrochemical reduction or hydrogenation of carbon dioxide and partial oxidation of lignocellulosic biomass.
We explore synthetic formate assimilation pathways that can be constructed using known metabolic enzymes as well as reactions that can be supported by the enzymes’ promiscuous activity. We focus on pathways with expected high biomass yield, high metabolic rate, favorable thermodynamics and a minimal number of enzymes.
We aim to implement the most promising pathways in heterotrophic, as well as photoautotrophic, organisms, thereby establishing an efficient formatotrophic growth and paving the way for the production of various value-added-chemicals from formic acid. We believe that such an approach could sustain large-scale biorefineries for a future in which our global chemical and energy industries could receive their building blocks solely from living organisms.
Redesigning photorespiration
For a new green revolution to feed the continually increasing population, agriculture productivity will have to be significantly improved. Photorespiration represents a big challenge in this respect, because it dissipates energy and leads to the futile loss of CO2, thereby limiting plant growth yield. Implementing an efficient metabolic bypass for photorespiration can therefore increase the photosynthetic efficiency of many cultivated crops. Several such routes were previously proposed. However, these routes were limited to existing enzymes and pathways and provided only partial improvement.
We aim to engineer photorespiration bypass routes based on novel pathway structures and enzyme chemistries. Using an in silico approach we were able to identify several promising bypass routes that could phonetically support up to 60% higher biomass yield per turn of the Calvin Cycle and up to 30% higher yield per ATP. Together with our partners, we work to implement these pathways in bacteria and plants, aiming to achieve significantly higher agricultural yields.
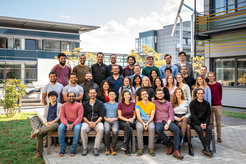